Dual-pinhole off-axis holographic 3D imaging system
In 1962, Leith and Upatnieks developed the off-axis holography which modulates the object term to higher frequency spectrum so that it can be easily extracted without any influence of the twin image term and zero order term. This greatly increased the signal-to-noise ratio (SNR) of the system. However, the system becomes much more complicated. Fig. 1(a) shows the system diagram of the off-axis holography. Two beam splitters are used to split and recombine the object wave and the reference wave. Mirrors, condensers and microscope objectives are used to precisely manipulate the wave in front of the object wave and the reference wave. The cost of the off-axis holography is so high that the commercial products are usually priced over 1 million RMB. Fig. 1(b) demonstrates one of the commercial product.
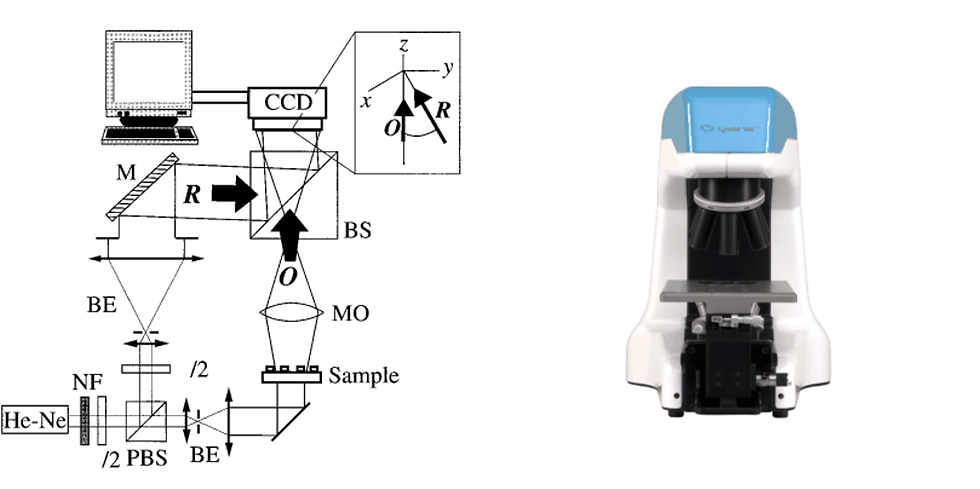
(a) (b)
Figure 1. (a) System diagram and (b) a commercial product of traditional off-axis holographic microscope.
To improve the image quality of previous two systems and lower the cost of off-axis holographic microscope, we developed a new system which combines the advantages of Gabor in-line holography and off-axis holography.
System setup
Fig. 2 shows the system diagram of our newly invented system which is called dual-pinhole off-axis holographic microscope. Except for the dual aperture, the geometry of our system is similar to Gabor in-line holography where the object is a few millimeters away from the point source and the digital light sensor is several times farther away. An inexpensive laser diode or RGB laser diodes casts a light spot on the dual aperture which produces two coherent spherical light sources. The first point source, emitted from the object pinhole, illuminates on the object, while the other one, emitted from the reference pinhole, illuminates on a clean area to create the reference wave. The two beams interfere with each other at the sensor plane to create a hologram which is then captured by the digital sensor. And instead of in-line Gabor holograms, off-axis holograms can be captured using the dual-pinhole system to reconstruct images with twin image eliminated.
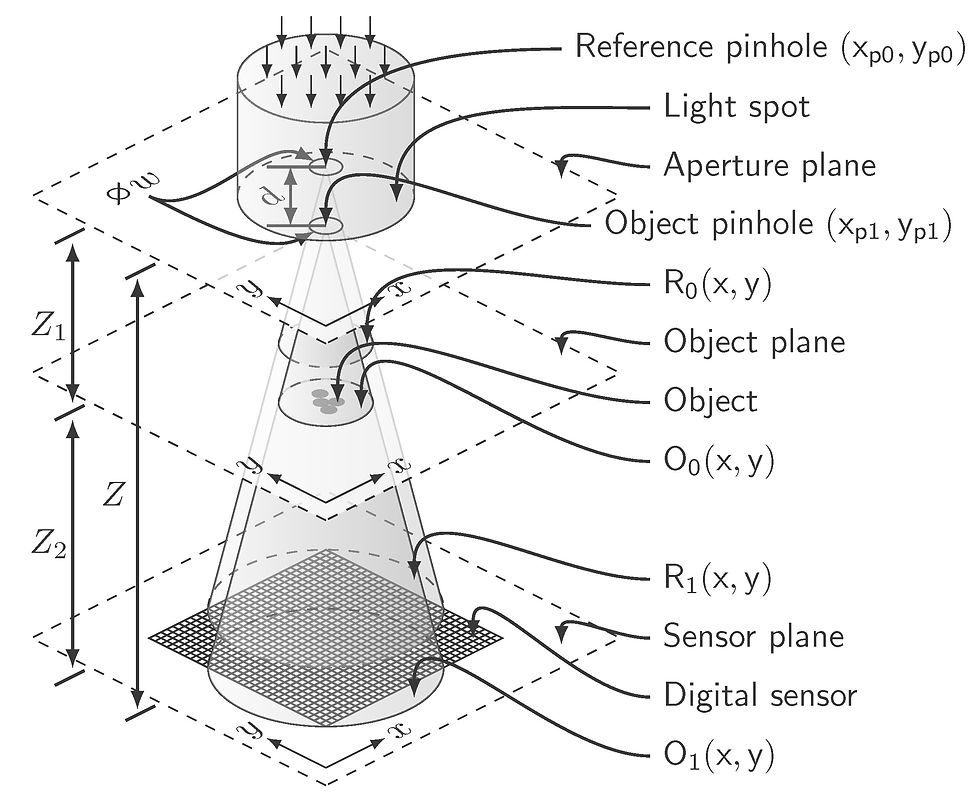
Figure 2. System diagram of the dual-pinhole off-axis holographic microscope
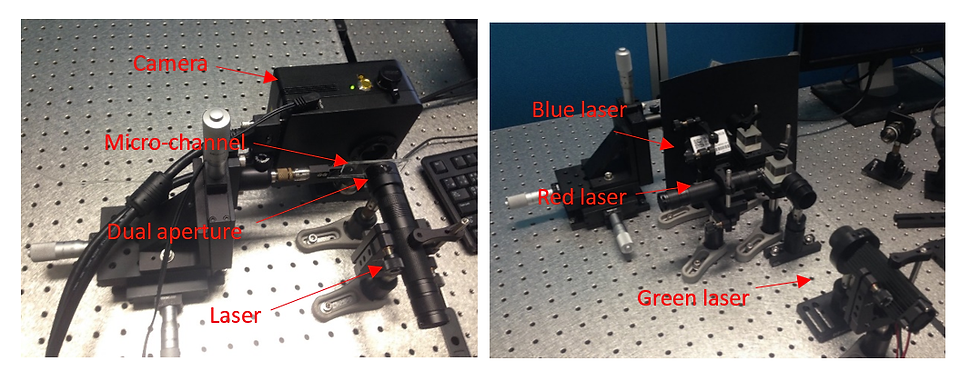
(a) (b)
Figure 3. The prototype of (a) grayscale system and (b) color system
Fig. 3 illustrated the experimental setup of the dual-pinhole off-axis holographic microscope. For the mono-color system, an inexpensive laser diode (LD-T650H00, = 650nm, measured power 120mW ) is used as the light source. A three dimensional moving platform is employed to hold the micro-channel. The high speed camera developed by our team is placed on the one dimensional moving stage which helps to adjust the distance between the dual aperture and the digital sensor. The dual apertures are customized from National Aperture. Currently, the system utilizes apertures with the diameters of and instead of designed in previous section because there are no factories providing dual apertures with such small diameter.
For the RGB system, three different lasers (LD-T650H00, = 650nm; LD-E405G08, = 405nm; LD-Z532X03, = 532nm.) are combined using two beam splitters acting as the light source. The other parts of the RGB system are the same with the mono-color system.
With the off-axis holographic microscopes we developed, plenty of different kinds of samples were observed to test the performance of the system. First, USAF 1951 was observed with the apertures of to test the resolution of the mono-color system. Fig. 4 shows the captured hologram and reconstructed amplitude image of the USFA 1951. It can be seen that the smallest elements of the group 7 of the USAF 1951 can be clearly resolved. This implies that the resolution of the system is better than which is in agreement with the theoretical values listed in Table 2. Thus we assert that the resolution of the system can reach with apertures.

(a) (b)
Figure 4. (a) Hologram and (b) 2D amplitude reconstruction of the USAF 1951 with apertures
Second, biological samples were tested using mono-color system. Fig. 5(a) shows the reconstructed phase image of the human steam cells. A picture captured using a traditional microscope is also provided for comparison. This proves that quantitative phase profile of the sample can be retrieved using the proposed system.
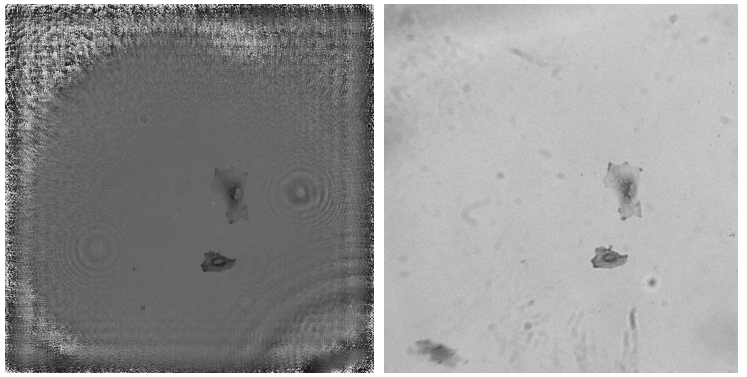
(a) (b)
Figure 5. (a) Reconstructed phase image and (b) picture captured using a traditional microscope of human steam cells.
Color system are also tested as shown in Fig. 6 where paramecia are used as targets.
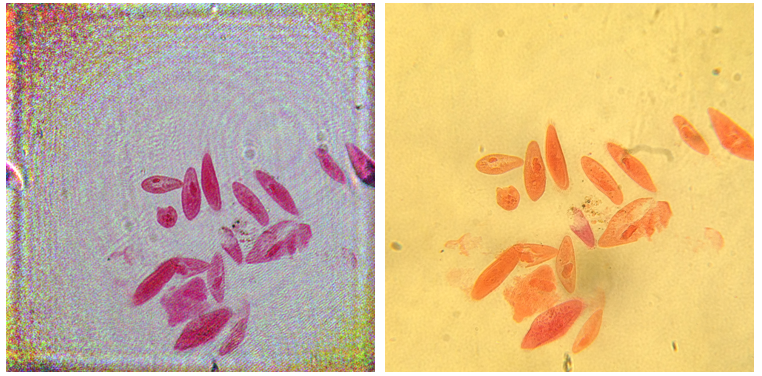
(a) (b)
Figure 6. (a) Reconstructed color image and picture captured using a traditional microscope of paramecia
In the end, several experiments were conducted to reconstruct 3D structure of the samples from the holograms captured using our system. Fig. 7 shows one of the results. A software were also developed to reconstruct the 3D point cloud of the sample in real time as shown in Fig. 8. With one core of GTX 690, the frame rate of program can reach ~40 Hz, so we predict that with two GTX 690s (totally 4 cores) the frame rate of the system can reach 150Hz. A paper based on the mono-color system with apertures was published in Optics Letters (the impact factor is 3.385).

Figure 7. 3D structure of a human stem cells

Figure 8. Real time reconstruction software